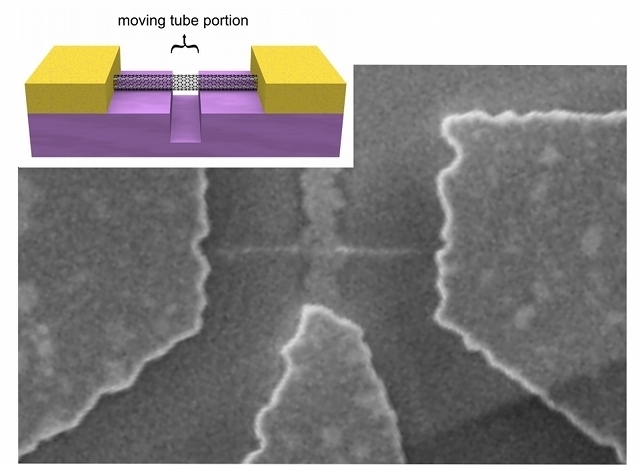
On macroscopic scales, we can use the force of gravity to help us determine mass. But on microscopic scales, other forces typically dominate. Nanoscopic mechanical resonators, which change their vibrational frequency in response to added mass, have allowed us to measure smaller objects, finding the masses of biological cells and large molecules. Now, researchers at the Universitat Autònoma de Barcelona have come close to the theoretical limit of these devices, building a nanomechanical resonator with mass resolution on the scale of individual protons.
In their Nature Nanotechnology paper, J. Chaste et al. describe a resonator built from a single carbon nanotube, one that is able to detect the presence of a small number of individual atoms added to it. The mass resolution they achieved is 1.7 yoctograms (1.7 yg, or 1.7 × 10-24 grams), which is the mass of a single proton. While devices of this sort aren't practical for measuring the properties of subatomic particles, they are extremely useful for atomic and molecular physics, as well as very sensitive mass spectroscopy.
The resonant device built by Chaste et al. is based on a single carbon nanotube approximately 150 nanometers (nm) long and 1.7 nm in diameter. Both ends are fixed, but its center is suspended over a narrow trench. (1 nm is 10-9 meters, or one billionth of a meter.) The nanotube has a resonant vibrational frequency of approximately 2 gigahertz (GHz), which is the source of its extreme sensitivity—even the slightest addition of mass will alter this resonance. The entire device is operated at about 6 degrees above absolute zero to isolate it from thermal vibrations that would interfere with the measurements.
As is typical in nanomechanical resonators, the nanotube is driven with an alternating electric current. When extra mass is added to the tube, its resonant frequency changes correspondingly: the adsorption of atoms and molecules on the tube's surface decreases the resonant frequency.
("Adsorption" refers to the binding of particles onto a surface, as opposed to "absorption," which involves incorporation into the interior of a material.)
Due to the extreme sensitivity of the nanotube resonator, Chaste et al. were able to detect the adsorption of individual xenon atoms and naphthalene (C10H8) molecules. These are approximately 100 times less massive than previous resonance experiments have achieved.
Additionally, the researchers were able to measure the binding energy of the xenon to the tube by determining how much the resonant frequency changed as the atoms depart from the surface. By comparing their results with what we know about xenon binding to graphite surfaces, they found that binding energy depends in part on the diameter of the nanotube. (Nanotubes are single sheets of graphite, also known as graphene, rolled into cylinders.) This has important implications for the storage of gases through adsorption.
Single nanotube resonators have great potential for measuring very small masses, allowing for extremely high precision in mass spectroscopy. But it's not only the mass; the precise location of the adsorbed particle also alters the resonant frequency. This means that, if we can get an impurity to a specific location on the nanotube, its mass may be measured directly, which has exciting implications for future research.
Nature Nanotechnology, 2012. DOI: 10.1038/nnano.2012.42 (About DOIs).
Listing image by Photograph by courtesy of Adrian Bachtold, Universitat Autònoma de Barcelona
reader comments
8